Apr 16, 2021 · 9 min read
Biology’s Most Ambitious Map Yet: What Is the Human Cell Atlas?
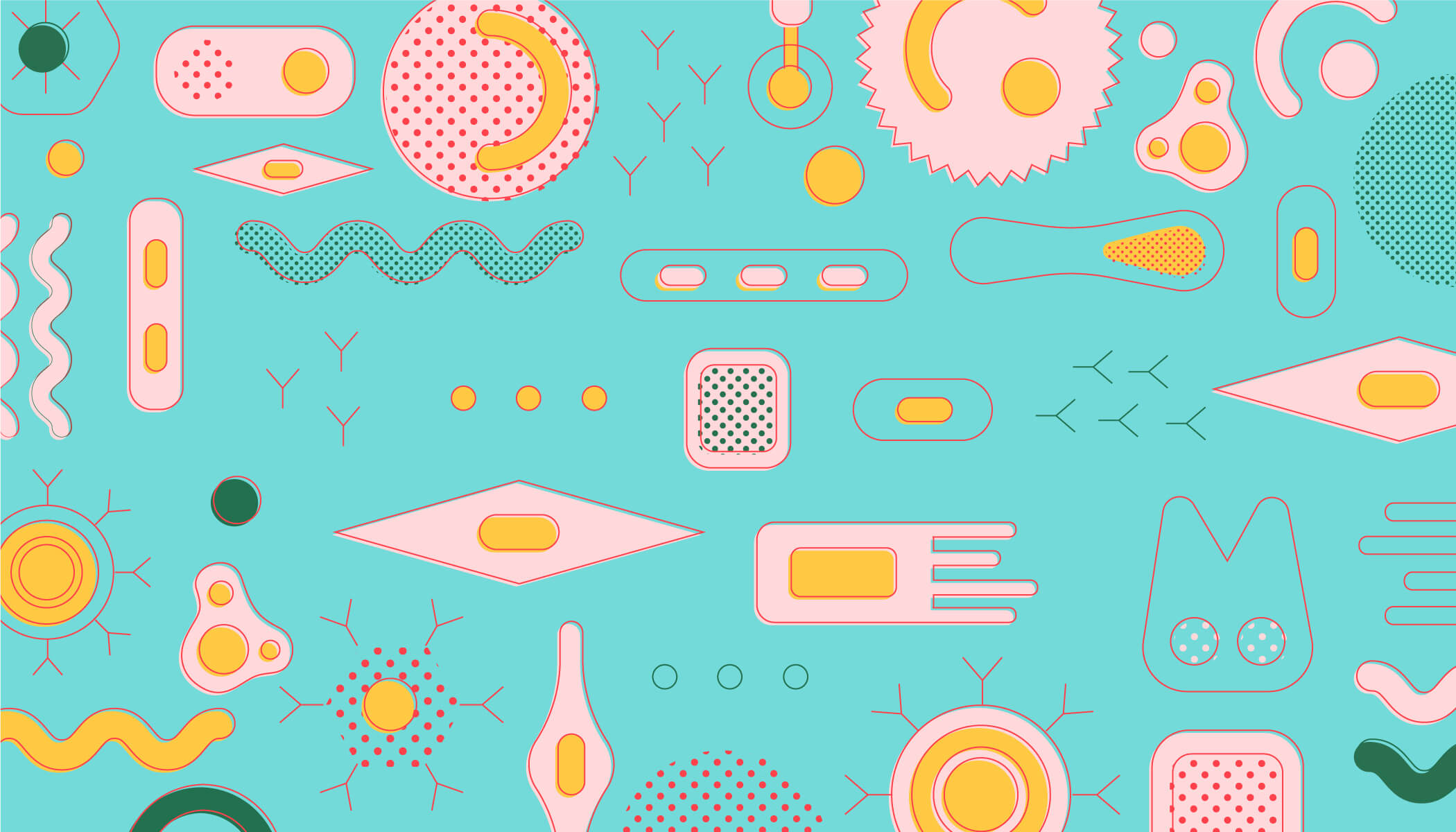
For more than two decades, using twin telescopes pointed skyward, scientists have been mapping the universe. From the outset, the trove of data they collect has been available to the scientific community and the public, fueling discovery and revolutionizing astronomy globally. Just last year, for example, the most comprehensive reference map of the cosmos was published, capturing images of more than three million stars and galaxies and spanning a mind-boggling 11 billion years of cosmic history.
Today, an equally ambitious effort is underway to map the wonders within us — the myriad of cells in the healthy human body. Known as the Human Cell Atlas (HCA), the project aims to create an open, shareable reference atlas of our cells, the building blocks of life. Its ultimate goal is to enhance our understanding of health and improve our ability to diagnose, monitor, and treat disease. After all, the roots of disease lie in our cells; observing the human body at this fundamental level has the potential to clarify all diseases.
Launched in 2016, the HCA is an international community of scientists focused on identifying and characterizing cells in different tissues, organs, and organ systems, including organs like the heart, lung, and kidney; and the reproductive, nervous, and immune systems. CZI was one of the first supporters of the HCA. Our focus has been on funding collaborative research teams that are developing new methods and generating data to accelerate progress toward a first draft of this cellular map.
There are about 37 trillion cells in the human body, made up of specialized cell types, such as immune cells that patrol for pathogens, cardiac muscle cells that rhythmically contract to pump blood, and pancreatic beta cells that secrete insulin. Scientists have a rough idea of the main cell types — most biology textbooks peg that number at a few hundred, but the reality is much more complex. Cells can look alike but behave differently; they can also change over time, in response to numerous internal and external signals. Importantly, cells don’t exist in isolation—they are part of an elaborate living quilt, carefully stitched together over thousands of years of evolution.
To date, HCA researchers have analyzed more than 39 million cells, revealing a menagerie of new rare and common cells.
To understand the human body in greater detail than ever before, a complete survey of healthy cells is urgently needed. Fortunately, the time is right. Just as bigger and better telescopes have allowed us to see deeper into the universe, an array of new technologies is revealing the secret lives of cells.
Leveraging the power of maps to understand the human body
Reference maps, or atlases, have underpinned progress in science for centuries. But they haven’t always gotten the recognition they deserve, in part because they are usually the product of slow and steady work by many researchers. Yet, the creation of reference maps has consistently pushed the boundaries of technology and of knowledge, laying the foundation for an incalculable number of future discoveries and innovations.
The Human Genome Project is a notable example. Led by an international team of researchers, it sought to map the genome, which includes all of the genes and many other regions that regulate them. The team published the first draft of the human genome 20 years ago, in February 2001, a half-century after the discovery of the structure of the DNA double helix. (The complete DNA sequence of the human genome, consisting of approximately 3 billion letters, was formally announced two years later, in April 2003). Former President Bill Clinton called it, “… the most important, most wondrous map ever produced by humankind.”
Science hasn’t been the same since. As the Human Genome Project decoded the book of life, it also helped rewrite the rules of research. The project’s scientists made a bold commitment to rapidly deposit the results of their research into a publicly accessible database. Those data were completely open, freely available to anyone. Today, easy access to genomic data has become the norm in biomedicine, and a broader movement toward open science has taken hold.
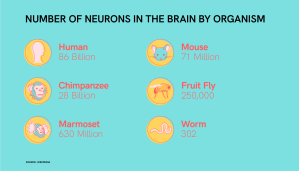
Beyond the human genome, some of the most impactful reference maps in biology describe simpler organisms, including yeast, bacteria, nematodes, fruit flies, zebrafish, sea urchins, and mice. Using these scaled-down systems, researchers can more readily unravel fundamental biological principles laid down by evolution that cut across the animal kingdom. They can also begin to answer some of the biggest questions in biology, such as: How do brain cells work together to generate thoughts, feelings, and actions? How does a complex animal develop from a single cell? And what goes wrong at the cellular level in diseases like cancer and cystic fibrosis?
The nematode Caenorhabditis elegans only has about 1,000 cells and grows to about one millimeter in length. Still, it holds an outsized place in scientific discovery. Why? Because back in 1986, researchers succeeded at mapping all of its 302 neurons and the 6,000 (or so) connections between them, creating the first complete wiring diagram of the brain in any species.
Sydney Brenner, who later shared the Nobel Prize in Physiology or Medicine in 2002, published the results in a landmark paper he playfully called “The Mind of a Worm.” Since then, generations of scientists have made progress toward understanding how the nervous system functions, using data from the open-source Worm Atlas. They have identified the neural circuits that drive complex behaviors like foraging, feeding, and mating, and the genes that orchestrate them, directly demonstrating how the brain controls behavior.
Scientists completed a more detailed version of the worm’s neural wiring in 2019, and efforts are now underway to create a complete connectivity atlas for the mouse brain, which has an estimated 71 million interconnected neurons. The ultimate goal is to understand how the mammalian brain is organized, and how its components work together to generate behavior.
Similarly, a reference map of sea urchins has also left its mark on biology. In the 1990s, scientists worked out the gene regulatory networks that transform a single-celled sea urchin egg into a multicellular organism. These networks provide the instructions for building a complex animal, telling embryonic cells what specialized cell types to become and where to move to form the sea urchin’s body plan — the anatomical structure a group of organisms shares as they develop. It turns out that many of the regulatory genes in these networks are universal, guiding the development of the body plan in other species, including humans.
A new toolkit for understanding the human body
The Human Cell Atlas continues this mapmaking tradition, but its researchers have a new — even revolutionary — toolkit at their disposal. Using technologies developed over the past decade, scientists can isolate thousands, if not millions, of cells from living tissue, look inside each one, and study their contents — including DNA, RNA, and proteins. This advancement unlocks levels of resolution in biology that connect genome maps to molecular maps, such as the sea urchin’s, to cellular maps, such as those of the worm or mouse.
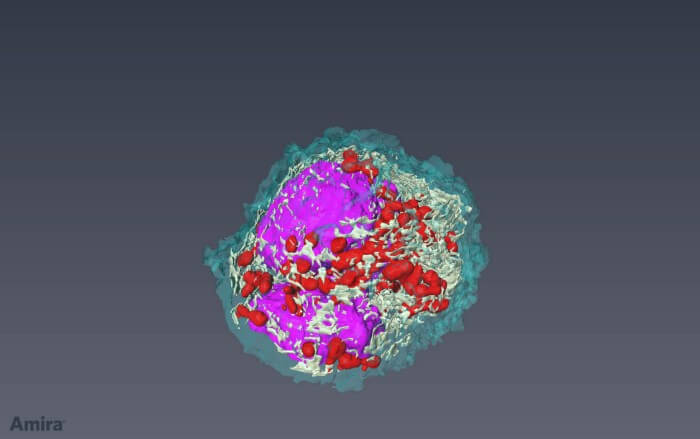
Single-cell RNA sequencing, or scRNA-seq, is the most widely used method in single-cell biology. It reads the RNA produced in a cell at a given time. That readout tells researchers which genes are active — and serves as a molecular blueprint for what a cell does.
The results from the first scRNA-seq study were published in 2009. Researchers can now use similar methods to read a cell’s DNA or proteins; or combine several techniques within a single cell. The results generate a unique molecular ID that distinguishes different cell types and provides a richer sketch of their functions.
The next step — one the field is already taking — is to study single cells in intact tissues rather than in isolation. Spatial technologies are helping researchers pinpoint the location of single cells within the living quilt — generating a three-dimensional map of the human body, including its most complex tissue, the brain.
The precision of these new techniques is extraordinary; so, too, is their speed and scale. Single-cell technologies can process hundreds of thousands of cells in a single experiment, making the goal of mapping all the cells in the human body realizable.
To date, HCA researchers have analyzed more than 39 million cells, revealing a menagerie of new rare and common cells. For example, they identified a new cell type in the airway that may be implicated in cystic fibrosis, a genetic disorder that causes persistent lung infections and progressively limits a person’s ability to breathe. Researchers also used HCA data to identify cells in the eyes and nose that may serve as the initial entry route for SARS-CoV-2, the virus that causes COVID-19. These results are already transforming our view of the human body.
As the data pour in, the HCA is also expanding, with support from CZI and other partners. For example, CZI is funding projects dedicated to mapping cells in healthy pediatric tissue to understand better how children’s biology differs from adults. A pediatric human cell atlas could deliver insights into childhood diseases, where the need for new treatments is particularly urgent.
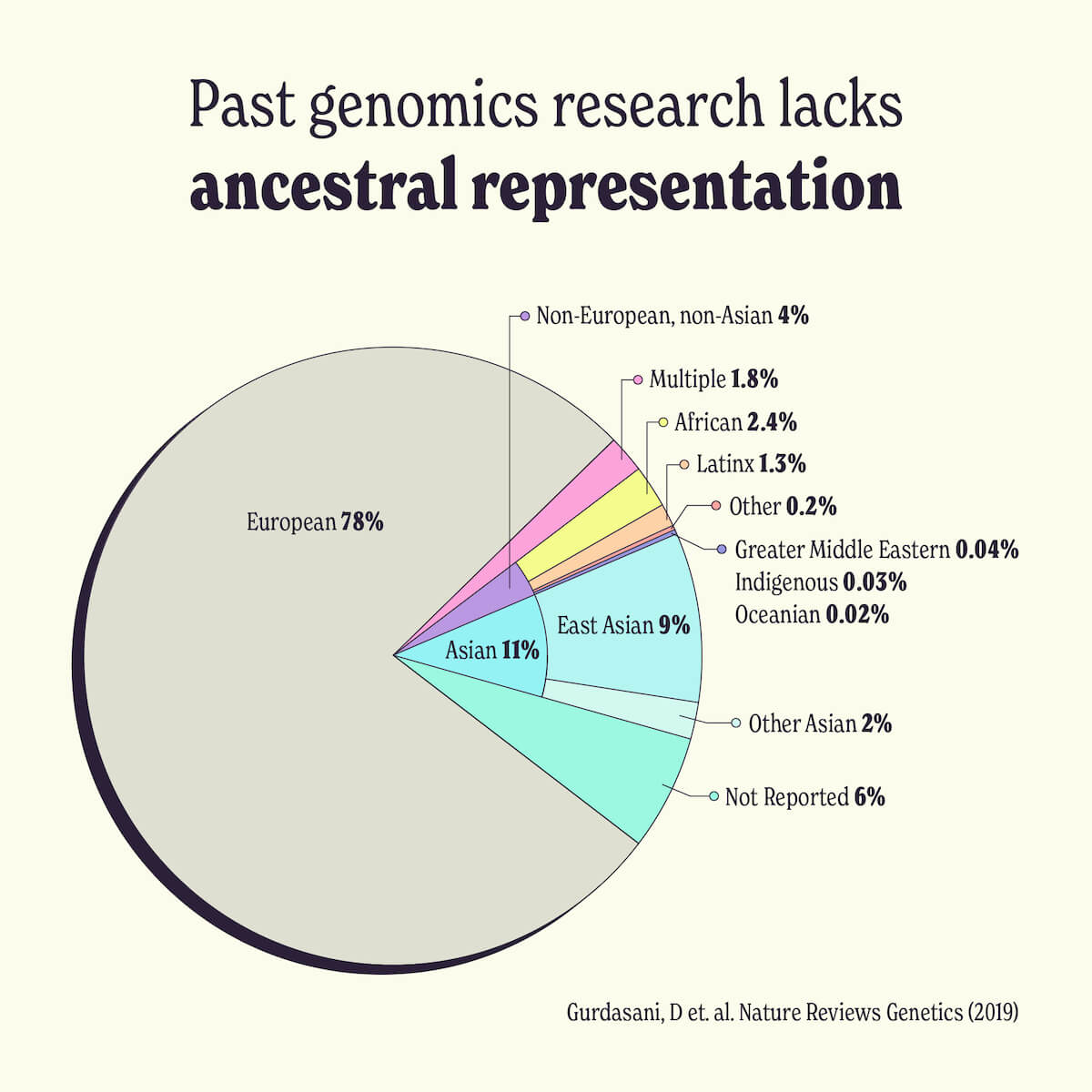
CZI is also funding a set of projects to expand the ancestral diversity of the samples in the HCA. Diverse tissue samples, including samples from Black, Latinx, Asian, and Indigenous people, are needed so that the results of the HCA benefit everyone. After all, diseases manifest differently in different people, and ancestry is one factor that impacts disease severity, outcome, and treatment. Black and Latinx people are at a higher risk for cardiovascular disease and respond differently to treatments. Understanding those differences at the cellular level could lead to better health outcomes for everyone, and references must be broadly representative, but in particular should include communities that bear the greatest burdens of disease.
Like the sky surveys and other reference maps that came before it, the HCA is poised to reveal the mysteries of the human body and spur new discoveries in biology and medicine for decades to come.