Nov 9, 2021 · 9 min read
Tracking How Childhood Diseases Develop at the Cellular Level
We need to understand what a disease is — down to the microscopic level of our cells — to better treat and manage it. And to prevent a disease altogether, we have to understand how it comes about so we can be ready to stop it in its tracks.
For childhood diseases, that means learning everything we can about prenatal and childhood development.
Researchers in the Pediatric Networks for the Human Cell Atlas are cataloging cells in healthy tissues from birth to late childhood. This will point them to clues to spot any changes in development that may lead cells and organs towards disease.
I am excited that dedicated research into child development is being undertaken. Research findings can directly help my patients and identify new treatments specifically for childhood diseases.
Learn more below about our grantees’ efforts to map cellular development and disease, from head to toe.
Examining Pediatric Skin Conditions: Skin Deep Is Actually Quite Deep
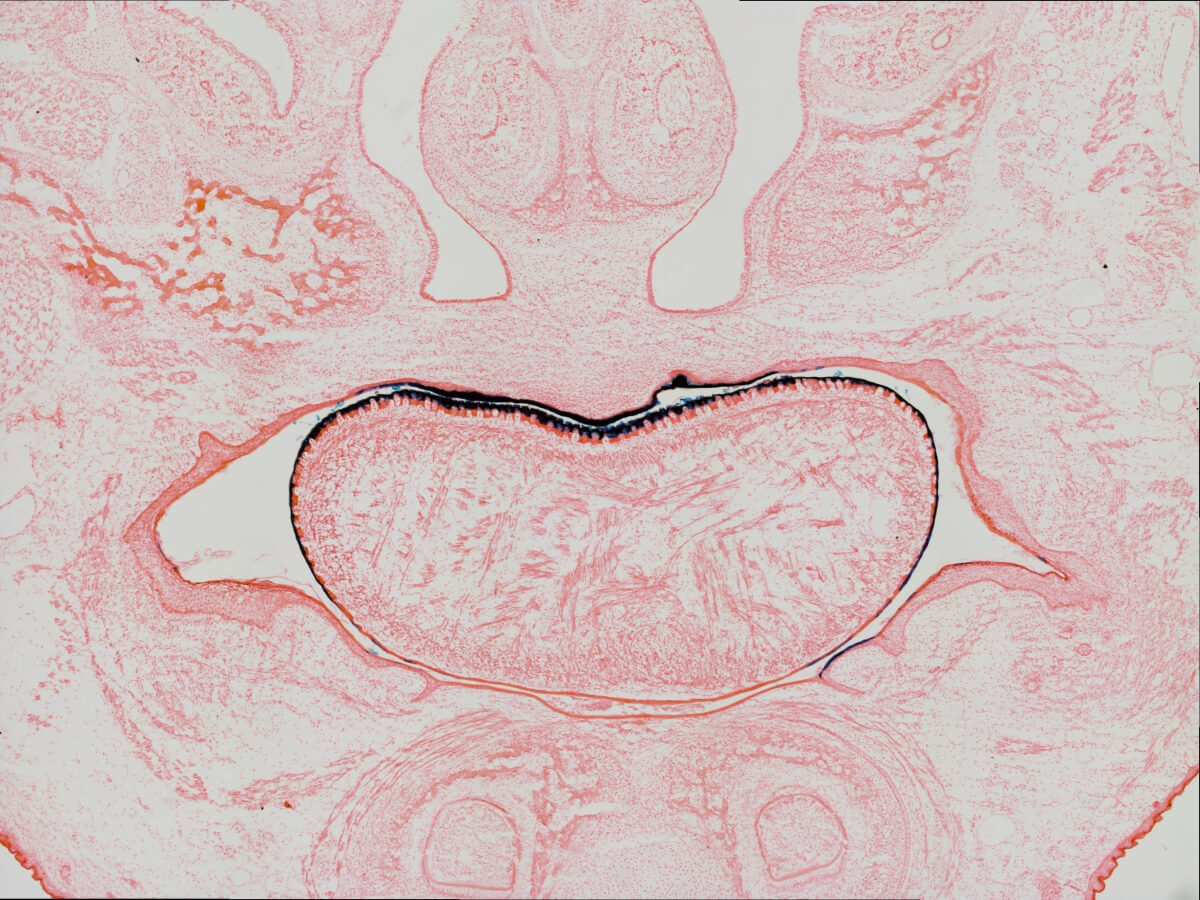
Healthy human skin develops across key milestones in our lives, starting from the day of birth when our skin has to adapt from the aquatic in-utero environment to a drier climate. Through this and other key developmental milestones, the skin changes and matures.
To learn more about the biological processes underpinning these changes, seven co-principal investigators from Wellcome Sanger Institute, Newcastle University and Queen Mary University of London (QMUL) in the United Kingdom are establishing an accessible repository of pediatric skin samples that will be shared freely to support other skin researchers and clinicians. This foundational work will help them learn more about pediatric inflammatory and infectious skin conditions, genetic skin diseases, and skin disorders specific to historically marginalized communities.
Among this team is Inês Sequeira, assistant professor at the Institute of Dentistry at QMUL. Inês brings to the team expertise from another related tissue, the cells lining the oral cavity — your cheeks, gums, tongue and inner lips — pictured above. The photo shows a section from top to bottom of a mouse head, showing the craniofacial structures and cells of the oral cavity. The tongue is the kidney bean-shaped structure in the middle.
The oral cavity is able to quickly regenerate with little scarring, properties also seen in younger skin tissue. By learning more about skin at different ages, this multidisciplinary Pediatric Networks team will gain insights about how skin develops in health and disease, inspiring innovative therapeutic strategies.
Studying Cells in the Nose of Children: The More You Nose
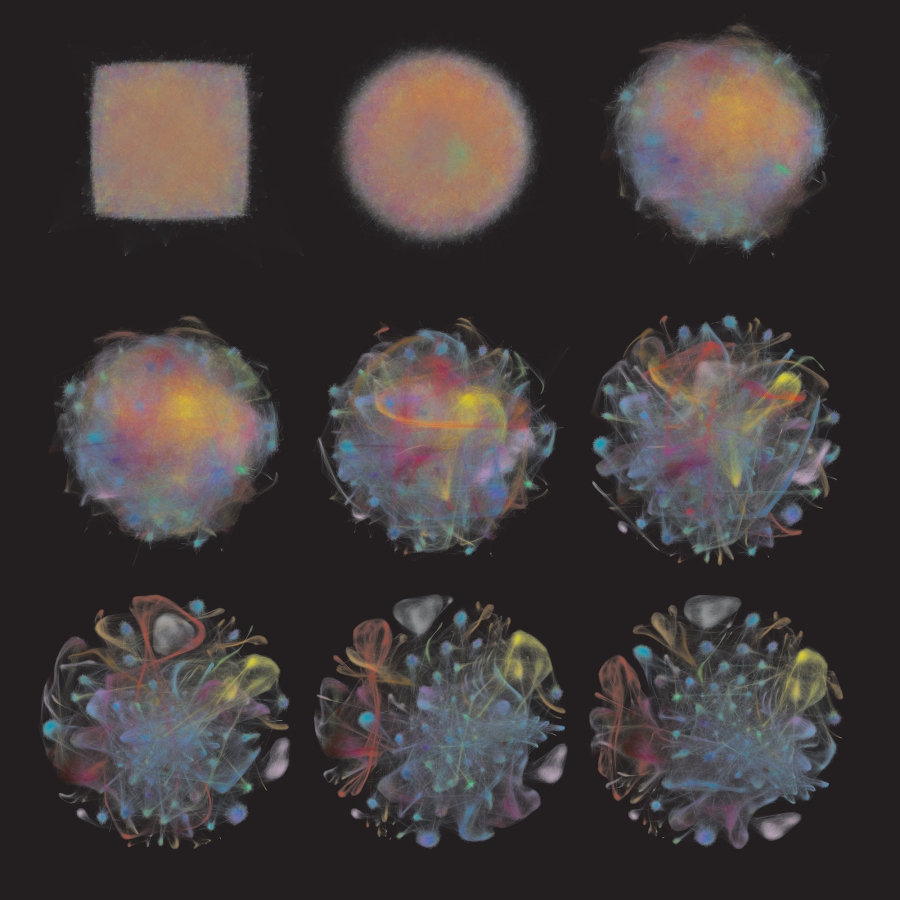
Your nose is a key interface between the inside of your body and the world around you. Its lining is a critical layer of defense and a primary site of many infections.
Jose Ordovas-Montanes, an assistant professor at Boston Children’s Hospital, is working with an international team of 10 other clinicians and researchers to create a reference map of all cells in the nose and chart how the interplay of genes and environment in this tissue during childhood maintain health or lead to allergy or disease in adulthood.
A quick and minimally invasive swab, similar to the ones used for COVID-19 PCR tests, can help researchers easily collect samples for this work. Then, they can extract genomic data from the swabs and visualize the similarities, differences and developmental relationships between cells.
In the photo above, Carly Ziegler (in Pediatric Networks grantee Alex Shalek’s lab) took this approach for cells from different tissues in the body. By applying this technique to cells from nasopharyngeal swabs, the team wants to expand the potential types of single-cell studies to understand diseases of relevance for communities around the world.
“We are working to overcome one of the central challenges in single-cell biology,” Jose says, “to increase the representation of scientists and research participants from around the world in genomics research from beginning to end.”
Adipose Tissue Cells: Fascinating Fats
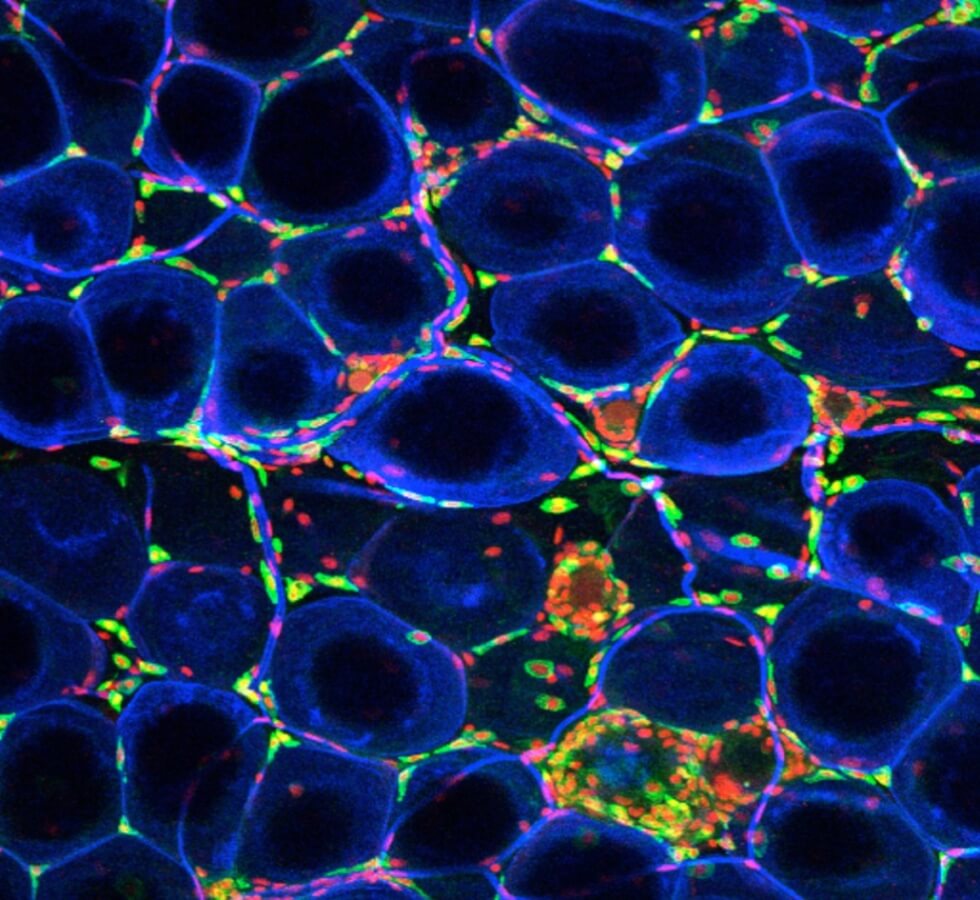
Fat, more formally known as adipose tissue, is an essential part of our bodies. Adipose tissue is central for growth, maintaining metabolic balance, regulating appetite, controlling body temperature, and regulating nutrients that change dramatically in early life and are important contributors to child and adult health.
Adipose tissue is made up of many different types of cells, shown above — from the fat cells themselves to immune cells and other structural cells. These cells differ based on the location of fat in the body, age, and body weight.
Carey Lumeng, a professor of pediatrics, is collaborating with colleagues at the University of Michigan Medical School to generate a comprehensive atlas of changes in adipose tissue in different areas of the body throughout childhood at single-cell resolution.
By better understanding how these tissues mature from childhood through adolescence and puberty, this team of Pediatric Networks grantees will gain a better understanding of how diseases rooted in dysfunction of adipose tissue, such as metabolic syndrome and Type 2 diabetes, begin in children.
“I am excited that dedicated research into child development is being undertaken,” says Carey. “Research findings can directly help my patients and identify new treatments specifically for childhood diseases.”
Also read: How Lab-Made Stem Cells Are Transforming Research
Pediatric Liver Biology: Nature’s Detoxifier, Decoded
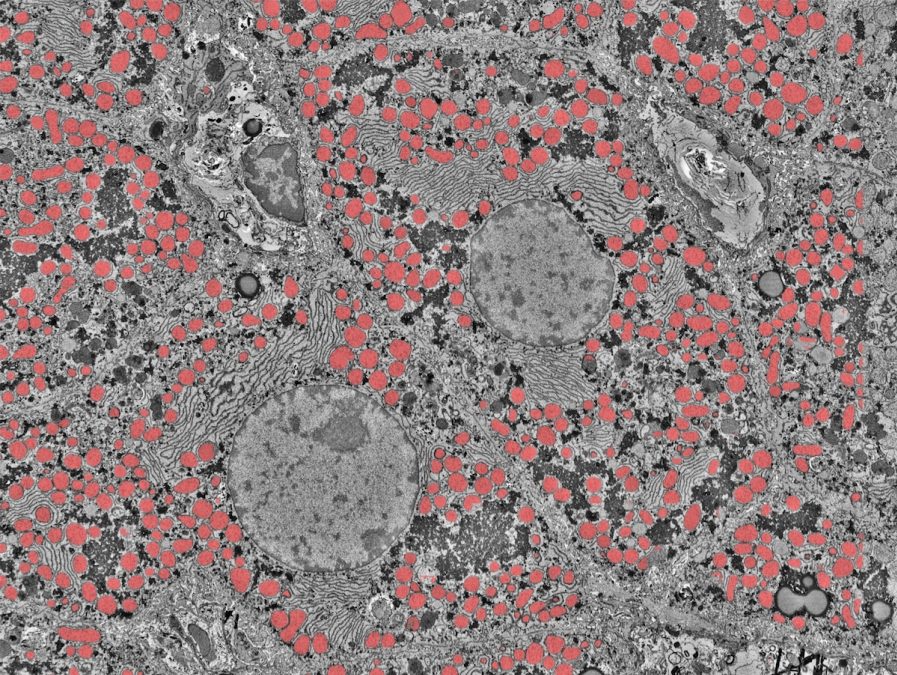
The liver is a powerful organ that drastically alters its functions during development. Shortly before birth, the liver transitions from being the main site of blood development and begins to take on its essential adult functions, like coordinating metabolism, aiding digestion, keeping blood healthy and cleaning our bodies from toxins. We have limited understanding of how the liver regulates these activities as our bodies increase in size and mature from birth to late childhood.
We tend to notice when the liver is dysfunctional because it can lead to noticeable symptoms like confusion, bleeding, jaundice and increased sensitivity to infection. But, we know less about how its many different types of cells come together to keep us healthy day to day.
To better understand this vital organ, a group of nearly 20 international collaborators in the Pediatrics Networks are mapping the healthy liver at single-cell resolution — throughout pediatric development and in diverse ancestries.
Above is an ultra-high resolution image of the human liver taken by electron microscopy from the labs of Gary Bader, Mei Zhen and Sonya MacParland. Gary, a professor at The Donnelly Centre at the University of Toronto, and Sonya, an assistant professor at the University of Toronto, are among this team of Pediatric Networks grantees. Computational methods are used to identify details in the image to better understand the difference between a healthy and diseased liver. For example, above the cell’s mitochondria are highlighted.
“Children are not little adults — their biology can be fundamentally different,” Gary says.” By figuring out how cells and tissues develop over childhood, we could potentially catch diseases in childhood, much earlier than usual, and prevent them from progressing in adults.”
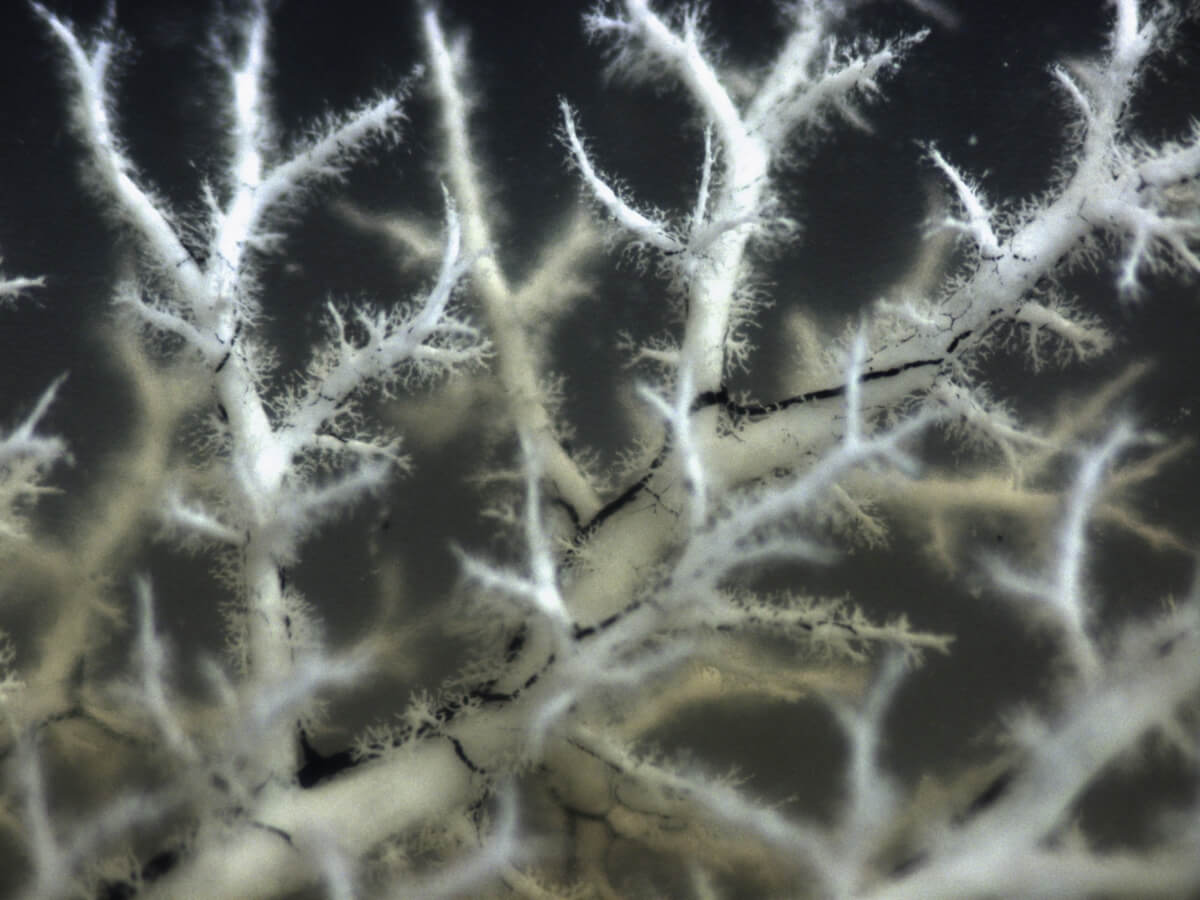
This image is a three-dimensional blueprint of the hepatic portal vein (white) and the bile ducts (black) from the lab of Stacey Huppert, an associate professor at Cincinnati Children’s Hospital Medical Center.
“For rare genetic pediatric liver diseases,” says Stacey, “information at the single-cell level will greatly expand our knowledge so we can target cell-specific common pathways to alleviate disease.”
Microbes and Intestinal Cells: The Gut Space-Time Continuum
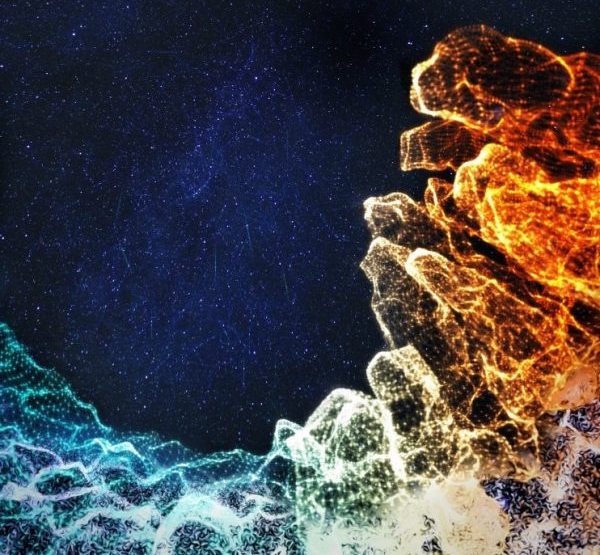
There is a whole universe of microbes and diverse cell types in your intestine. During childhood, the intestine typically develops a healthy immune system in symbiosis with its many microbial inhabitants.
But somewhere during this development, if that balance is disrupted, it can lead to inflammatory bowel diseases like Crohn’s disease and ulcerative colitis.
A trio of investigators from the University of Oxford previously provided open access atlases of the human colon in health and inflammatory bowel disease and a resource documenting how the human fetal intestine develops. Now, they are building on this work to better understand how a healthy immune system develops in symbiosis with intestinal microorganisms.
Hashem Koohy, an associate professor of systems immunology at the University of Oxford and part of this team, shared the artistic rendering above. It represents the team’s work to build a detailed map of the developing intestine showing its changes over space and time in neonatal, to childhood and adult diseases.
Pediatric Gut Cell Biology: The Inner Atlas
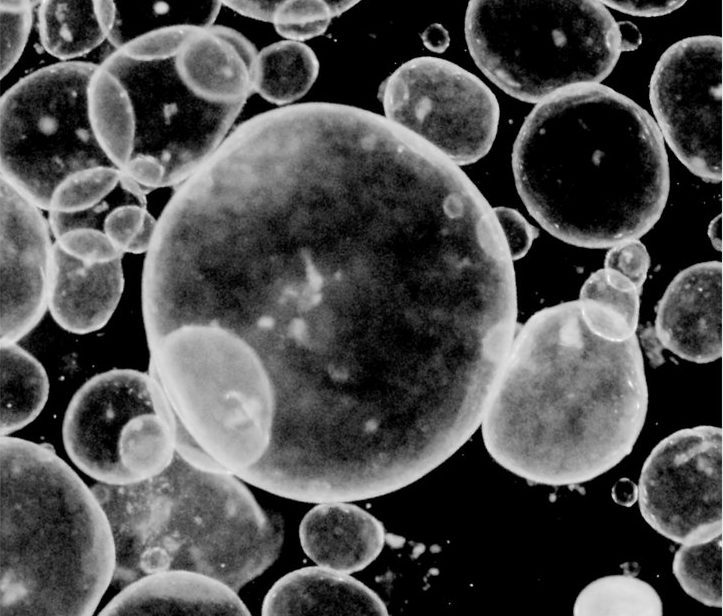
If we want to interrupt the development of disease, we need to map the full disease timeline. But for many childhood diseases, this process may begin before birth, making it hard for researchers to observe the earliest stages of disease formation.
To overcome this, an international group of researchers in the Pediatrics Networks grow mini organoids in a dish, derived directly from patient biopsies or pluripotent stem cells, to study the development of respiratory and digestive tissues. The photo above shows gut organoids from the lab of Jason Spence, professor at the University of Michigan Medical School.
Cells of the respiratory and digestive tracts are cousins in the developing embryo since they’re both made from an earlier embryonic layer of cells called endoderm. By building a pediatric cell atlas of the developing endoderm, this Pediatric Networks team will fill in the gaps of how the respiratory and digestive tracts develop and progress into disease.
“The entire pediatric cell atlas initiative is hugely exciting,” says Jason. “It will open up many previously unexplored aspects of human development, tissue maturation, and our understanding of disease.”
Explore our website for more about the research we’re funding for the Pediatric Networks for the Human Cell Atlas.
Editor’s note: This story was last updated on December 14, 2022.