Nov 29, 2022 · 8 min read
How Lab-Made Stem Cells Are Transforming Research
Learn how lab-made stem cells are powering biomedical research and leading to breakthroughs in our understanding of human biology.
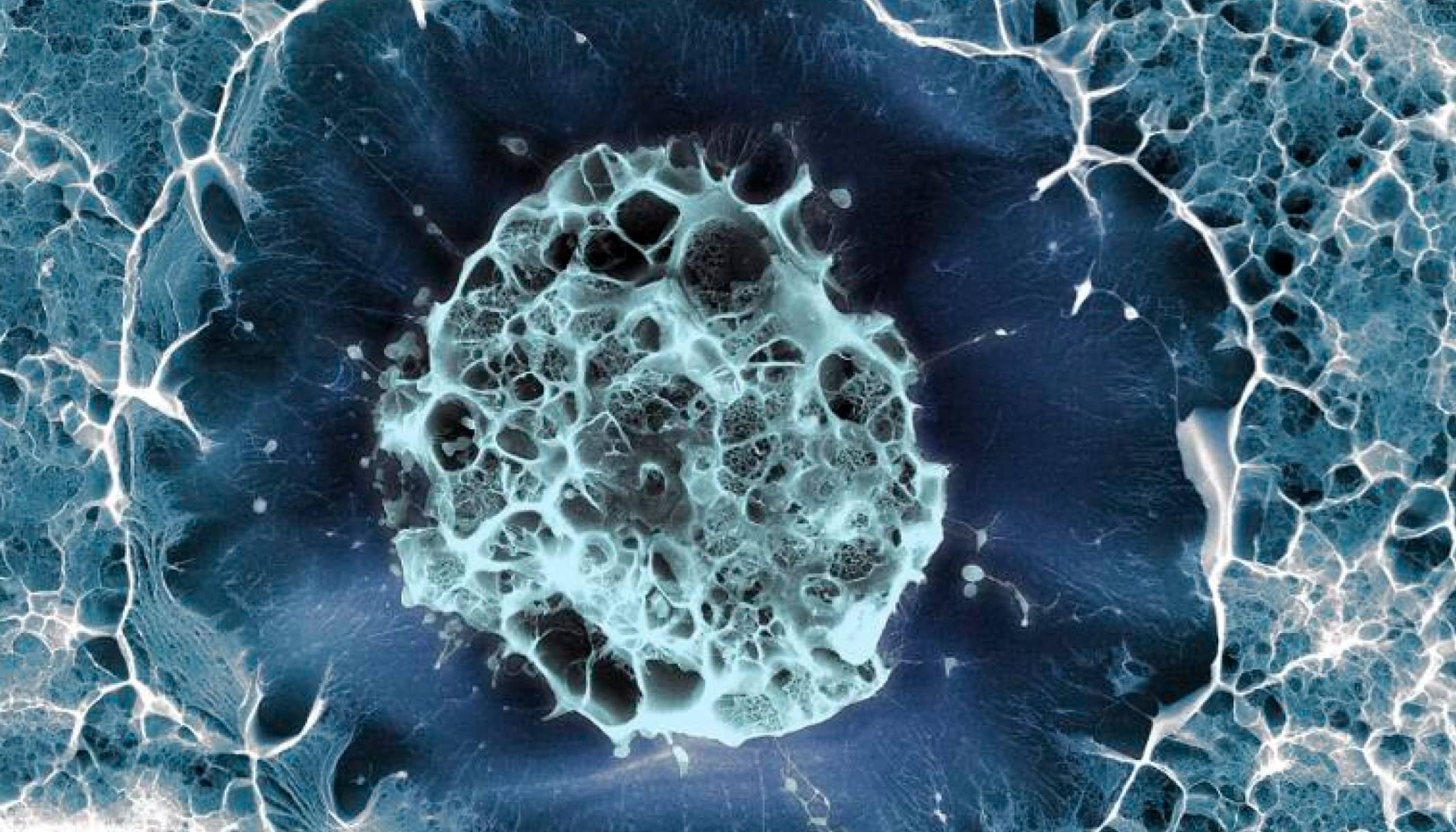
There are cells within our bodies that have a spectacular power. Known as stem cells, they build our bodies before we’re born and regenerate our tissues to keep us alive. For example, stem cells in the skin help us heal from cuts and other stem cells deep in the bone marrow replenish our blood and immune cells.
There are two defining characteristics that make stem cells special: they can make copies of themselves — a process called self-renewal; and, they can mature into more specialized cells — a process known as differentiation.
These properties make stem cells living laboratories for medical research, helping scientists study human biology in action from the earliest stages of development. In the lab, they allow us to model the cellular roots of disease and test the effectiveness of new drugs. The cells could also serve as living therapeutics for the treatment of life-threatening diseases, such as cancer, diabetes and Parkinson’s. The insights they yield will help accelerate progress toward curing, preventing or managing all diseases by the end of the century.
While stem cells have been fascinating to biomedical researchers for over a century, there was one Nobel Prize-winning finding that accelerated the field in the last two decades: the ability to reprogram mature cells into stem cells in the lab.
Also read: What Is the Human Cell Atlas?
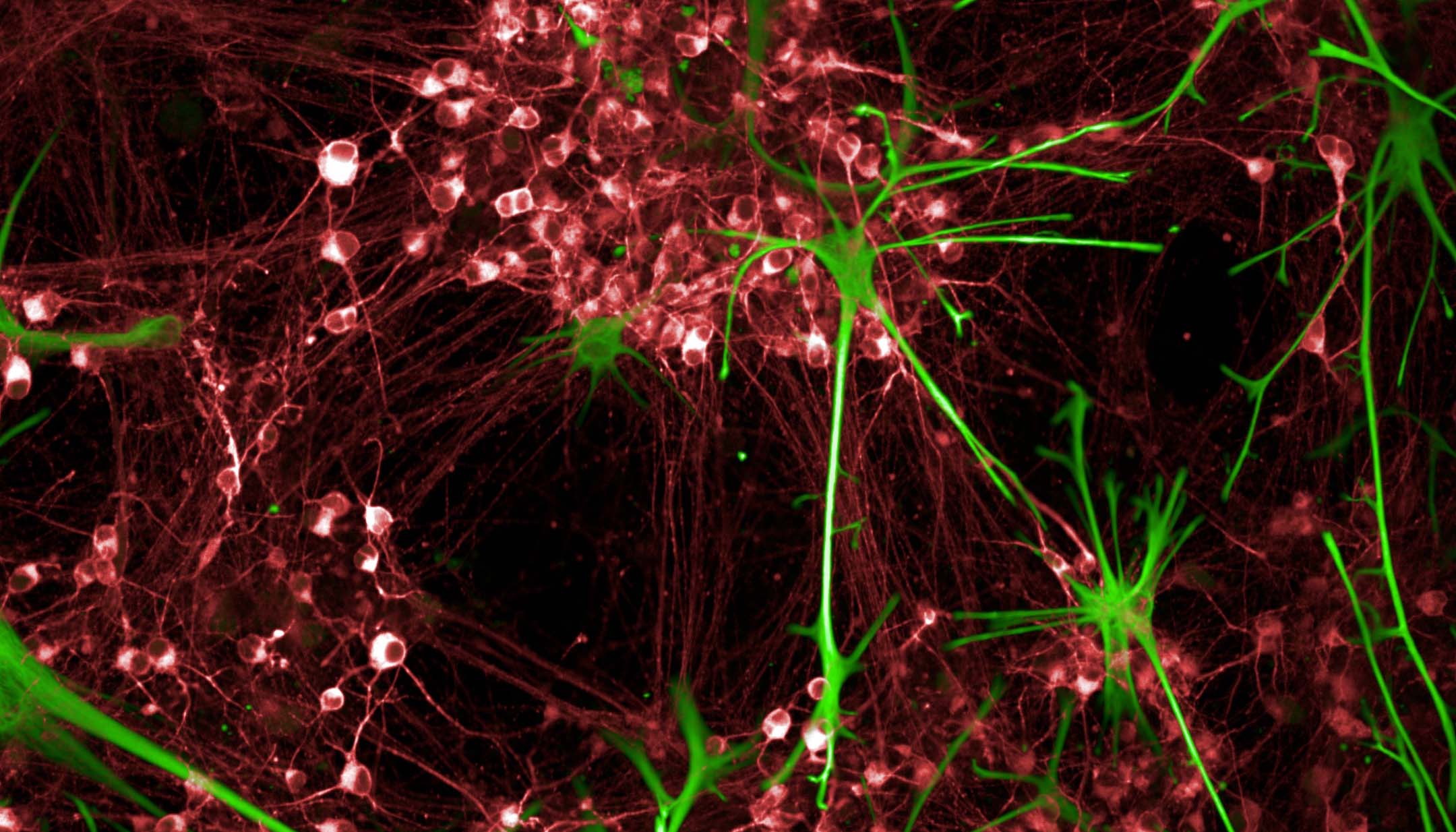
The Discovery of Induced Pluripotent Stem Cells
While stem cells exist in tissues throughout the adult body, stem cells in places like the brain, muscle and gut are not readily accessible for research. That changed in 2006, when scientists Shinya Yamanaka and Kazutoshi Takahashi created the first induced pluripotent stem cells (iPSCs, for short).
Shinya and Kazutoshi were able to make these “lab-made” stem cells by applying just four growth factors to ordinary mouse skin cells. These four factors were enough to reset the mature mouse skin cells. Within two to three weeks, the skin cells were transformed into one of the most powerful types of stem cells: pluripotent stem cells. Within the next year, Shinya’s lab and others showed this same method worked for human skin cells, too. Shortly afterward, labs across the stem cell research community systematically tested reprogramming on mature cells from throughout the human body.
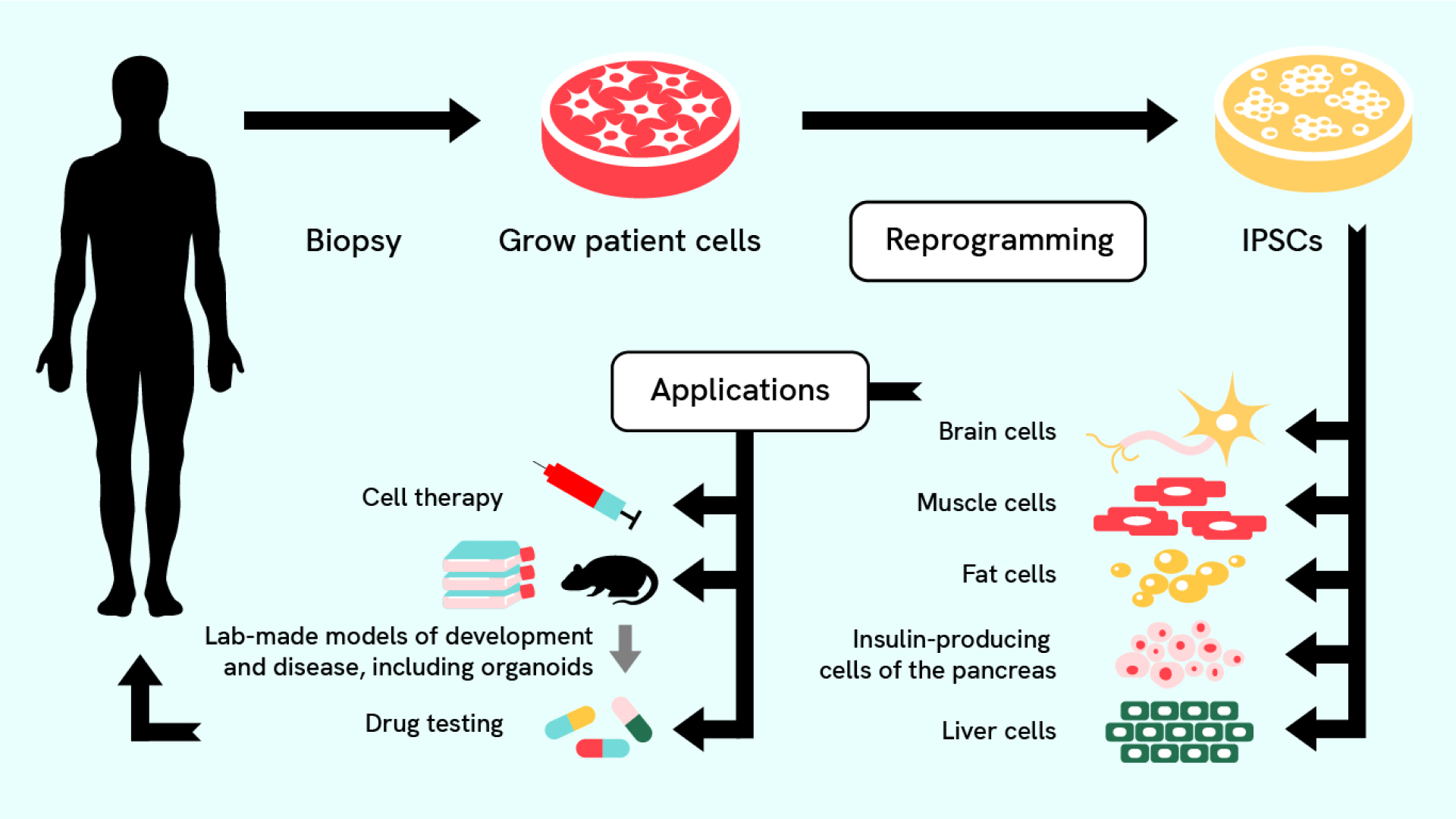
Prior to this discovery, pluripotent stem cells were only found in the earliest stages of development. Unlike the stem cells found in adults that usually only make cells from their tissue of origin, pluripotent stem cells can make almost any cell in the body (hence the name: “pluri” means many, like plural). The four growth factors added are highly active in stem cells of the early embryo, and, they later learned, are key to maintaining the cells’ ability to develop into various types.
Essentially they found a way to send mature cells — cells that were thought to be locked in their fate as skin — back in time to a more potent, embryonic-like state that can make any cell in the body. The discovery brought about a paradigm shift in biology and made it possible, for the first time, to study any cell in the body regardless of the available donor tissue. A mere six years later, Shinya, who led the research team, was awarded the Nobel Prize in Physiology or Medicine in 2012 for the discovery of iPSCs.
A New Era of Stem Cell Research
Over the past few decades, iPSCs have powered biomedical research. Under the right laboratory conditions, iPSCs can renew forever, and scientists have optimized making them in the lab and transforming them into diverse cell types. To coax them into forming specialized cell types, such as neurons, insulin-producing cells, or heart cells, researchers add growth factors or small molecules into a Petri dish.
For example, with the right signals, iPSCs can grow into neurons with their unique shape, structure and ability to send electrochemical messages to communicate with other cells. Research cataloging cells in development helps inform what those ideal signals are. Once researchers have plenty of new neurons on hand, they can use them to study diseases and may ultimately develop new disease treatments. In fact, a major drive is underway to use iPSCs to solve the mystery of Alzheimer’s and other neurodegenerative diseases. To support this effort, CZI helped fund the development of a warehouse of iPSCs. Scientists can order cells and use them to study the genetic changes that lead to Alzheimer’s disease, amyotrophic lateral sclerosis (ALS), and several other disorders.
Some of these cell types are grown in a two-dimensional culture dish, just like in biology class. Others are grown in three dimensions, forming multicellular clusters called organoids that resemble regions of intricate tissues like the brain and kidney. Organoids are more realistic models for researchers studying the human brain or other human tissue.
A Brief History of Induced Pluripotent Stem Cells
Explore the timeline below to learn about the history of iPSCs.
Organoids: When Stem Cells Go 3D
Three years after the discovery of iPSCs, scientists used them to create the first organoids, miniature three-dimensional clusters of cells. These mini models were a major step forward in stem cell research because they are more representative of the three-dimensional structures cells that form in the human body. Tissues contain multiple cell types, which are organized in a specific way and form connections to function together. By watching cells develop and mature in an organoid, researchers can study some of the tissue characteristics that are seen early in human development.
For example, there are mini 3D models of human intestines that give researchers a front-row seat to gut development and lung organoids that are helping scientists figure out which cell types are infected by SARS-CoV-2 and how the immune response damages the lung.
There are also brain organoids — 3D models of brain tissue grown in a dish. Some brain organoids resemble the developing cerebral cortex, the complex, multi-layered structure making up the outer surface of the brain. Others mimic the spinal cord or the cerebellum, the fist-sized brain region at the back of the head that coordinates movement. Brain organoids give researchers the unique opportunity to study live models of brain tissue like never before.
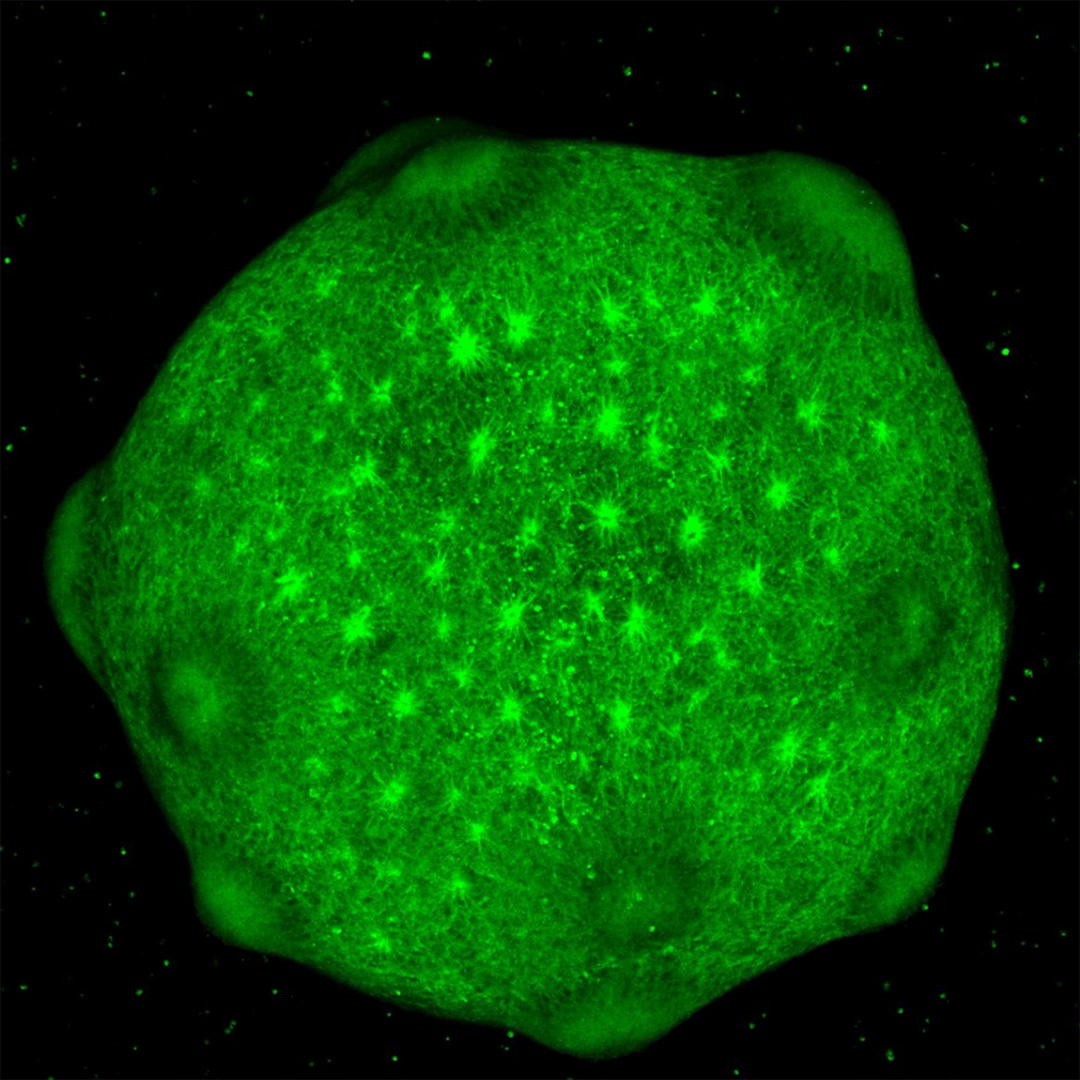
In 2019, about a hundred cortical organoids traveled to the International Space Station so that scientists could study the effects of microgravity on human brain development.
In 2021, CZI grantee Sergiu Pasca and his collaborators reported that they had kept brain organoids alive for nearly two years to study how they change over time. They found that after 250–300 days, the cells undergo biological changes similar to those seen in the developing brains of newborn babies. In 2022, Pasca published work showing that transplanting organoids made from human iPSCs into a rat brain could help them model brain circuits.
The science of organoids is still young, but researchers are finding ways to grow them for longer periods of time and achieve more complexity and maturity than ever before. Like iPSCs grown in two dimensions, they are being used to study organ development, model disease, screen for new drugs, and produce essential molecules like insulin. They may eventually be used to repair organs.
The Future of Stem Cell Research
Breakthroughs in stem cell research have made a clear impact, yet much work in this field of science remains. For example, iPSCs are not an exact copy of stem cells made by nature. Scientists are trying to figure out why — and whether the differences really matter for research and medicine. Researchers are also still seeking to better understand how reprogramming works, how to reliably grow all the different cell types in the human body, engineer cells to be immune-compatible, assemble cells into representative organoids, and massively scale up the production of both cells and organoids.
At the same time, scientists are getting tantalizingly close to realizing the dream of regenerative medicine — the ability to restore diseased and injured tissues and whole organs. While iPSCs carefully march toward the clinic, these wondrous cells will continue to help researchers study the human body in a way that wasn’t possible until a little more than a decade ago. And they will continue to reveal the fundamental biology that builds and maintains us.
Read our How It Works series to learn about other science and technology breakthroughs. Learn more about how CZI supports research on neurodegeneration, including disease-specific stem cell lines.